Research Themes
TADF and HLCT emitters
The major challenges to be considered while designing organic emitters are listed below:- The organic emitters should have high photoluminescence quantum yields, which directly impact the device efficiency.
- The energy levels of the frontier molecular orbitals (i.e., highest occupied and lowest unoccupied molecular oribitals (HOMOs and LUMOs)) of each of the layers in the device should be precisely aligned to: i) minimize the barrier to charge injection, and ii) control the recombination region within the device, which greatly affects both the device efficiency and lifetime.
- The organic materials must demonstrate sufficient thermal stability to be compatible with their vacuum deposition during device fabrication or produce thin films of suitable morphology when spin-coated during solution processing.
- Regardless of the fabrication method, the organic material must be morphologically stable during device operation when Joule heat is produced in the device.
Another key issue to address is the management of hole and electron recombination within the device, each possessing their own spin. Unlike photoexcitation, in which mainly singlet excited states are produced in the organic emitters, exciton formation through charge (hole and electron) recombination in OLED devices results in 25% singlets and 75% triplets, according to spin statistics. Whilst the singlets contribute to light emission by fluorescence (kF), with short emission lifetimes (in the nanosecond regime), phosphorescence (kP) from the triplets with emission lifetimes extending to milliseconds occurs due to the spin-forbidden nature of the emission. The very long emissionmlifetime makes the triplet excitons vulnerable to nonradiative deactivation as heat loss to the surroundings. Assuming Lambertian emission and a light outcoupling efficiency of 20%, the maximum external quantum efficiency (EQE) for an OLED with a fluorescent emitter is only 25% of 20% which is 5%.
In 1998, Baldo et al. reported an OLED device comprising red-emitting porphyrine complex doped into a fluorescent host which exhibited 23% IQE, owing the harvesting of both singlets and triplets. Triggered by this seminal contribution pohosphorescence based OLEDs employing organometallic complexes, mainly iridium complexes, as emitters have been developed with nearly 100% IQE. However, the rarity of the heavy-metal precursors is a major challenge to cutting down the cost of the devices and also pose environmental contamination of heavy metals which are cause of health concern. Though the organometallic complexes faired satisfacotily in red and green-emitting devices, the blue-emitting complexes lacked color purity and required brighness. The poor performanc eof blue phosphorescent OLEDs are due to triplet-polaron annihilation which results in the formation of highly energetic polarons what cause device degradation and unstable radical cations which cause ligand dissociation. So at present, the major challenge is the design of deep-blue stable emitters.
An answer to this challenge was presented in 2011 by utilizing an organic thermally cativated delayed fluorescent (TADF) material. Similar to phosphorescent emitters, TADF emitters can harvest both singlet and triplet excitons and hence achieve 100% IQE. The advantage with TADF emitters is that they can be purely organic, thus avoiding the health concerns associated with heavy metals.
TADF depends on the singlet-triplet energy gap, ΔEST, defined as the gap between the lowest energy triplet state (T1) and the lowest energy singlet state (S1). If ΔEST is sufficiently small (<0.1 eV), then the thermal upconversion from the triplet state to the singlet state by reverse intersystem-crossing (RISC) is possible. When the S1 and T1 states are dominated by HOMO to LUMO transitions, ΔEST is highly dependent on the electron density overlap between the HOMO and LUMO. As per El-Sayed's rule, ISC (and RISC) to efficiently occur there must be a change in the symmetry of the excited state. ISC/RISC will be fast if, for instance, T1 were a locally excited (LE) state while S1 were a charge-transfer (CT) state. Excited states involved in the TADF process are not typically either pure CT or LE states but mixed CT-LE with possibly different fractions of CT character. Thus, qualitatively, in order for an organic material to display TADF emission, it should have the following characteristics:
- Well separated HOMO and LUMO orbitals. Reducing the overlap between the HOMO and LUMO decreases the overlap integral and hence ΔEST
- S1 and T1 states should have different fractions of CT charater to allow ISC/RISC as per El-Sayed's rule
- Presence of heavy atoms such as sulfur may assist ISC/RISC by heavy atom effect
Organic Solar Cells
In order to secure the energy supply of the future several alternate energy resources have been explored. One promising technique is the direct conversion of sunlight to electrical energy using photovoltaic cells. Conventional photovoltaic cells are manufactured using rare semiconductors and metals. Their material properties are untunable by chemical modifications. These factors combined with their high cost make them unsuitable for large area deployment.
In recent years, solar cells fabricated using organic materials gained immense attention. The promise of this new class of organic solar cells (OSC) lies in their flexibility and potentially very low production costs. Organic photovoltaics (OPV) have achieved efficiencies surpassing 11%, but efficiency limitations as well as long-term reliability remain significant barriers for further applications.
OPV cells use molecular or polymeric light-harvesting materials, which results in a localized exciton on absorption of light. This electron donor is used along with an electron acceptor, such as a fullerene derivatives PCBM, which possess molecular orbital energy states that facilitate electron transfer. Upon absorbing a photon, the resulting exciton migrates to the interface between the donor material and the electron acceptor material. At the interface, the energetic mismatch of the molecular orbitals provides sufficient driving force to split the exciton and create free charge carriers (an electron and a hole).
The physical properties of organic donors, such as band gap and absorption spectrum, can be tailored by tuning their chemical structure through controlled synthetic reconstructions and careful choice of functional entities. They normally display high molar extinction coefficients, therefore thin layers (~100 nm) of them are sufficient to harvest light. Also, they can be processed at room temperature using solution which makes them cheaper.
Some of the merits of OSC are listed below:
- Cost effective renewable energy solution
- Composed of abundant and non-toxic elements such as carbon, nitrogen and sulfur
- Solution processable thereby roll-to-roll manufacturing friendly
- Tunable transparency, color and flexibility
- Versative applications
The low efficiencies of OPV cells are related to their small exciton diffusion lengths and low carrier mobilities. These two characteristics ultimately restrict the use of them as thin active layers that affect overall device performance. Current research focuses on increasing device efficiency and lifetime. Substantial efficiency gains have been achieved already by improving the light-harvesting material.
The efficiency of the OSC is dependent on the following proceses:
- Photon absorption by the photoactive layer
- Exciton migration to, and charge transfer at the interface
- Separation of the charges from their mutual Coulomb attraction
- Transport of separated charges to the electrodes.
The substitution of fullerene derivatives that absorb only a limited fraction of the sunlight with alternative acceptors is a rational approach to maximize the absorption of the photoactive layer. Perylene diimide (PDI) dyes are a prominent class of novel acceptor materials that have recently shown promising power-conversion efficiencies up to 4% in combination with low bandgap polymers[1] and small molecules.[2] Besides strong absorption in the visible perylene diimides offer advantages e.g. good electron transport properties, versatile synthetic possibilities to tune energy levels, solubility as well as stacking behavior, and very good thermal and photo stability.
Our research on OPV falls under the following categories:
- New materials design and synthesis
- Materials for all small molecule devices
- Non-fullerene acceptors
Non-fullerene Acceptors
Since the first demonstration of bilayered organic solar cell (OSC) in 1986, optimization of the performance of OSC has been studied in detail. Their efficiency has been incremented from the initial 1% to the current 10-12% through either donor-material or cell-structure explorations. There was only a limited scope for functional tuning of fullerene derivatives. (see below)
OSC is typically made from a blend of organic semiconducting polymer such as P3HT and fullerene-based acceptors such as phenyl-C61-butyric acid methyl ester (PC61BM) of phenyl-C71-butyric acid methyl ester (PC71BM). These fullerene acceptors were derived from the parent C60 and C70 fullerenes, by incorporating suitable groups, to improve the solubility and processability. Fullerene derivatives are advantageous due to their (i) ability to accept and transport electrons in three dimensions thanks to a LUMO that is delocalized over the whole surface of the molecule, (ii) high electron mobilities, (iii) multiple reversible electrochemical reductions, and (iv) ability to aggregate in bulk heterojunctions to form both pure and mixed domains of the appropriate length scale for charge separation.
Nevertheless, fullerene-based acceptors have some limitations including (i) weak absorption in the visible region of the incident solar spectrum, which limits their ability to harvest photocurrent, (ii) limited tunability in terms of spectral absorption by chemical modification, (iii) high synthetic costs, especially for the high performing C70 derivatives, and (iv) morphological instability due to fullerene diffusion and aggregation in the thin film over time.
Due to these reasons, recently, there is a growing interest in the development of new non-fullerene electron acceptor (NFA) materials.
New NFAs should obviously retain the advantageous properties of the fullerenes, such as efficient charge transfer with a low possibility of back transfer and good blend morphology with the donor material. They should also display greater ease of synthesis, improved solubility and processability from green solvents, increased optical density and introduce a structural flexibility that allows for favorable matching of frontier energy levels of donor and acceptor materials.
NFAs that are slightly weaker electron acceptors than fullerenes. They are likely to work well with wide band gap donors such as poly(3-hexylthiophene-2,5-diyl) (P3HT) due to the diminished LUMO-LUMO offset (ΔLUMO) affording a higher open-circuit voltage (VOC). On the other hand, the availability of stronger electron acceptors than fullerenes is important when considering narrow band gap donors, which often perform poorly with fullerene acceptors due to an insufficient LUMO-LUMO offset.
We at IIT Roorkee are involved in the development of new electron acceptors which can be used with both wide and narrow band gap donors in OPV.
Nitrogen-rich Heterocycles
Energetic materials are solid, liquid or gaseous substances - or mixtures of these - which are capable of very fast chemical reactions without the use of additional reactive species (e.g. oxygen). The reaction can be initiated by means of mechanical, thermal or shock wave stimuli. Generally the reaction products are gaseous. Energetic materials can be applied in explosives, rocket and gun propellants, pyrotechnics, gas generators etc.
Funding Sources
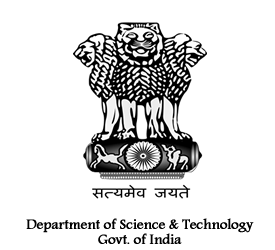
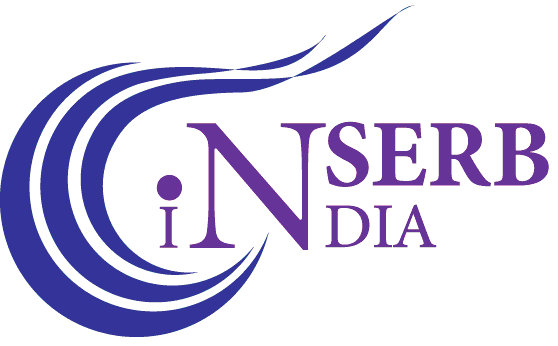
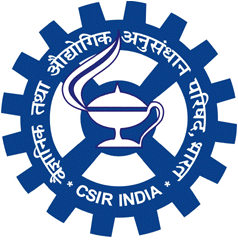
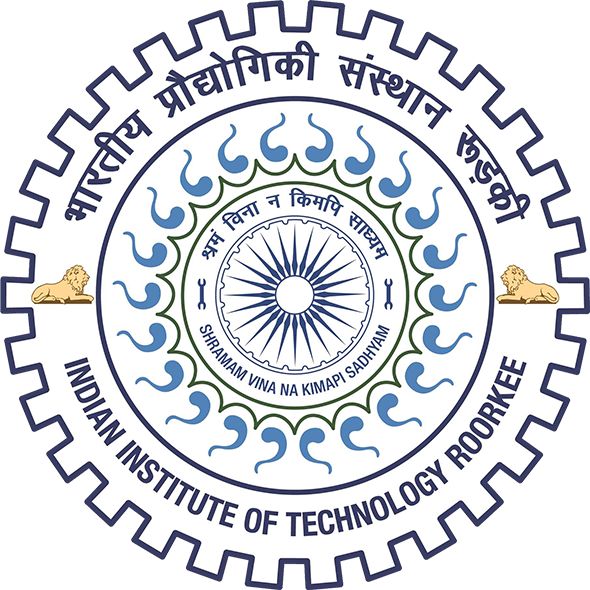